50 Years Ago: The First Look at a Dry Mars
In 1964, Caltech astronomy professor Guido Münch and Jet Propulsion Laboratory space scientists Lewis Kaplan and Hyron Spinrad pushed the world's second-largest telescope to its limits and dashed—at least for the next few decades—any hopes of finding liquid water on Mars.
Back in the late 1800s, it was widely assumed that Mars was a planet with abundant water, just like Earth. Astronomers were mapping Mars's polar caps, which advanced and retreated as the seasons changed; a dark "wave," apparently of vegetation, which swept from the pole toward the equator every spring; and even ruler-straight lines that might have been canals dug by an alien civilization. Today, we know that the ice caps grow larger because the winters are cold enough to freeze carbon dioxide right out of Mars's thin air; the seasonal darkening is a wind-driven redistribution of the dust that blankets the planet; and the canals were optical illusions enhanced by wishful thinking.
The notion of a moist Mars began to evaporate at the turn of the 20th century. In 1909, Lick Observatory dispatched a team of astronomers to climb Mount Whitney—whose summit, at 14,500 feet, rises above some four-fifths of Earth's atmospheric water vapor. Pointing a small telescope at Mars, the team measured no water vapor in excess of that in the rarefied air around them, although observatory director William Wallace Campbell cautioned the Associated Press that their technique, "the only method known, is not a sensitive one." Campbell diplomatically noted that "the question of life under these conditions is the biologist's problem rather than the astronomer's."
Bigger telescopes make for more sensitive measurements, and by the 1920s the world's largest telescopes were just north of Pasadena at the Mount Wilson Observatory. In 1926, observatory director Walter Adams and Charles St. John wrote in the Astrophysical Journal that "the quantity of water-vapor in the atmosphere of Mars, area for area, was 6 per cent of that over Mount Wilson . . . This indicates extreme desert conditions over the greater portion of the Martian hemisphere toward us at the time." The 60-inch telescope they used was second in size and power only to the adjacent 100-inch Hooker telescope, with which Adams revisited the question in 1937 and 1939 and revised his figures downward. In 1941 he wrote, "If water vapor lines are present . . . they cannot be more than 5 per cent as strong as in the earth's atmosphere and are probably very much less."
The "lines" Adams referred to are spectral ones. The spectrum of light contains all the colors of the rainbow, plus wavelengths beyond, that we can't see. Every gas in the atmosphere—both Earth's and Mars's—absorbs a specific collection of these colors. Passing the light from a telescope through a device called a spectrograph spreads out the rainbow and reveals the missing wavelengths, allowing the gases that absorbed them to be identified.
In those days, spectra were usually recorded as shades of gray on glass plates coated with a light-sensitive emulsion—essentially the same technique photographer Matthew Brady had used to document the Civil War. Once the plates were developed, the missing wavelengths showed up as black lines that were painstakingly analyzed under a microscope. Each line's location indicated its wavelength, while its darkness and thickness were related to the absorber's abundance. And therein lay the problem: the wide, black blots left on the plate by Earth's dense blanket of air made the thin, faint lines from the tenuous atmosphere of Mars hard to see, let alone measure. The best opportunities to find the lines occur at approximately two-year intervals. Earth travels in a tighter orbit around the sun than Mars does, and as we pass Mars on the inside track our close approach maximizes the apparent difference in our velocities. This shifts Mars's spectrum ever so slightly away from Earth's—if you have an instrument powerful enough to discern the separation.
Unfortunately, some passes are closer than others. When Earth overtook Mars in 1963, the latter was at the point in its orbit most distant from the sun. Although the two planets were as close to each other as they were going to get that time around, the velocity effect was minimized—imagine looking out the window of a moving train at a distant farmhouse instead of the nearby telephone poles. But the Hooker's spectrograph had recently been upgraded; Kaplan and Spinrad were expert spectroscopists; and Münch was a wizard at making very sensitive emulsions, so the trio decided to look for the lines anyway. With little prospect for success, the experiment was allotted a set of low-value nights that began more than two months after Earth had passed Mars and started to pull ahead. At its closest approach, Mars had been 62,000,000 miles away; by the time Münch and company got their turn at the telescope, that distance had nearly doubled. Their telescope was no longer the best available, having been overtaken as the world's largest by the 200-inch Hale telescope at Caltech's Palomar Observatory. Even the weather conspired against them; four nights of work yielded exactly one usable exposure.
But as Münch wrote in the January 1964 issue of the Astrophysical Journal, that "strongly hypersensitized" plate gave "a spectrogram of excellent quality which shows faint but unmistakable lines which have been ascribed to H20 in Mars' atmosphere . . . After comparing our plate with other ones found in the Mount Wilson files, we have convinced ourselves that ours is the spectrogram of Mars with the highest resolving power ever taken."
Even so, the lines were barely strong enough to be usable. The preliminary water-vapor calculation, announced in May 1963, had an error factor of 10. It would take another six months to work out the definitive number—a figure equivalent to 0.01 ± 0.006 per cent of the amount of water vapor over Mount Wilson, and 100 times less than the 6 percent Adams and St. John had referred to as "extreme desert conditions" 40 years earlier. Furthermore, a slightly stronger carbon dioxide line enabled a direct estimate of Mars's atmospheric pressure: 25 millibars (2.5 percent of Earth's surface pressure)—one-quarter of the best previous estimates. (Munch and his collaborators noted in passing that although their value for carbon dioxide was not itself surprising, "what would appear indeed surprising is that the . . . value for the atmospheric pressure [is] so low that CO2 itself becomes a major constituent"—entirely unlike Earth, where nitrogen and oxygen make up 99 percent of the air we breathe.) Based on these results, Mars was now officially as arid as the moon, and nearly as airless.
Confirmation would follow in 1965, when JPL's Mariner 4 became the first spacecraft to visit Mars. The behavior of Mariner's radio signal as the spacecraft passed behind Mars revealed that its actual atmospheric pressure was lower still: 5 to 9 millibars, or less than 1 percent of Earth's. And the 20 televised pictures of Mars's cratered, moonlike surface—some shot from as little as 6,000 miles above it—cemented the comparison.
Professor of Physics Robert Leighton (BS '41, MS '44, PhD '47), who had been the principal investigator on Mariner 4's Television Experiment, as it was called, and Associate Professor of Planetary Science Bruce Murray, a member of the TV team, would use Münch's and Mariner's data as cross-checks on a detailed thermal model of Mars that they wrote for Caltech's IBM 7094 mainframe computer—a pioneering feat in its own right. Their results, published in 1966, correctly predicted that most of Mars's carbon dioxide was actually not in the atmosphere, but instead lay locked up in the polar caps in the form of dry ice; the paper also made the unprecedented suggestion that seasonal advance of each polar cap would freeze out so much carbon dioxide that the atmospheric pressure would drop by as much as 20 percent twice every Mars year. These predictions have since been confirmed many times over, and form part of our basic understanding of how Mars works.
And what of water on present-day Mars, which is where this story began? Leighton and Murray wrote that "considerable quantities of water-ice permafrost may be present in the subsurface of the polar regions" just a few tens of centimeters down—permafrost that was finally discovered in 2002 by JPL's Mars Odyssey mission.
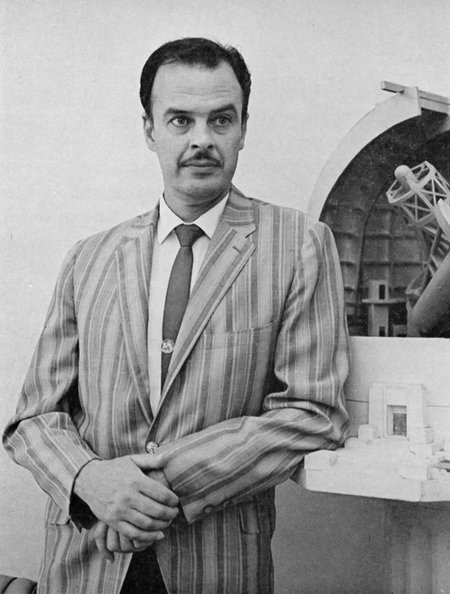
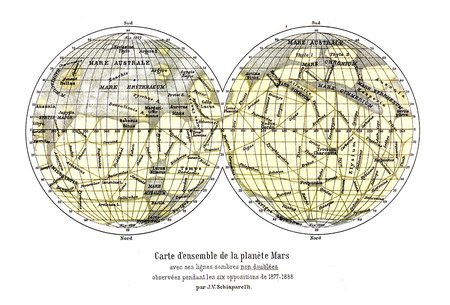
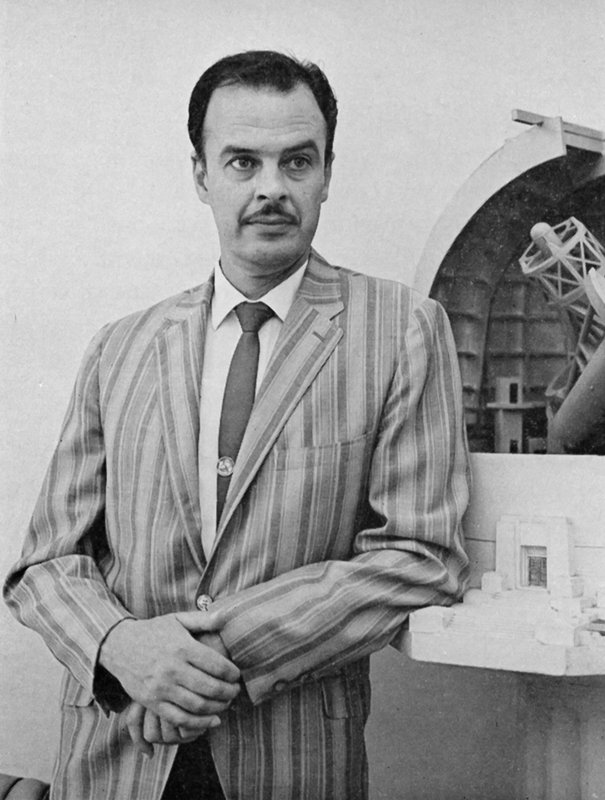
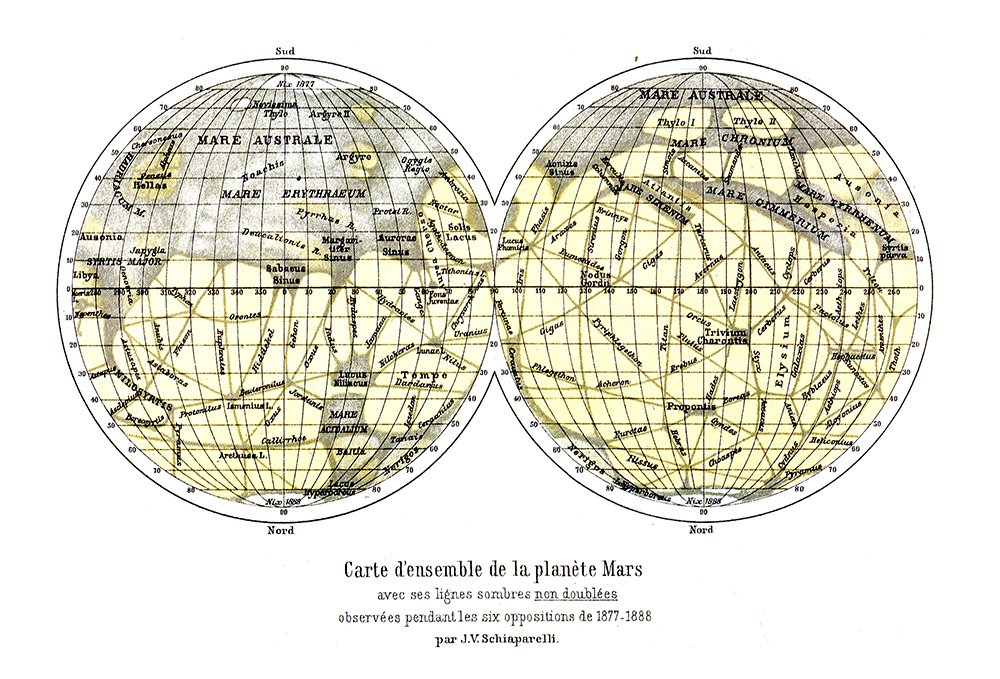