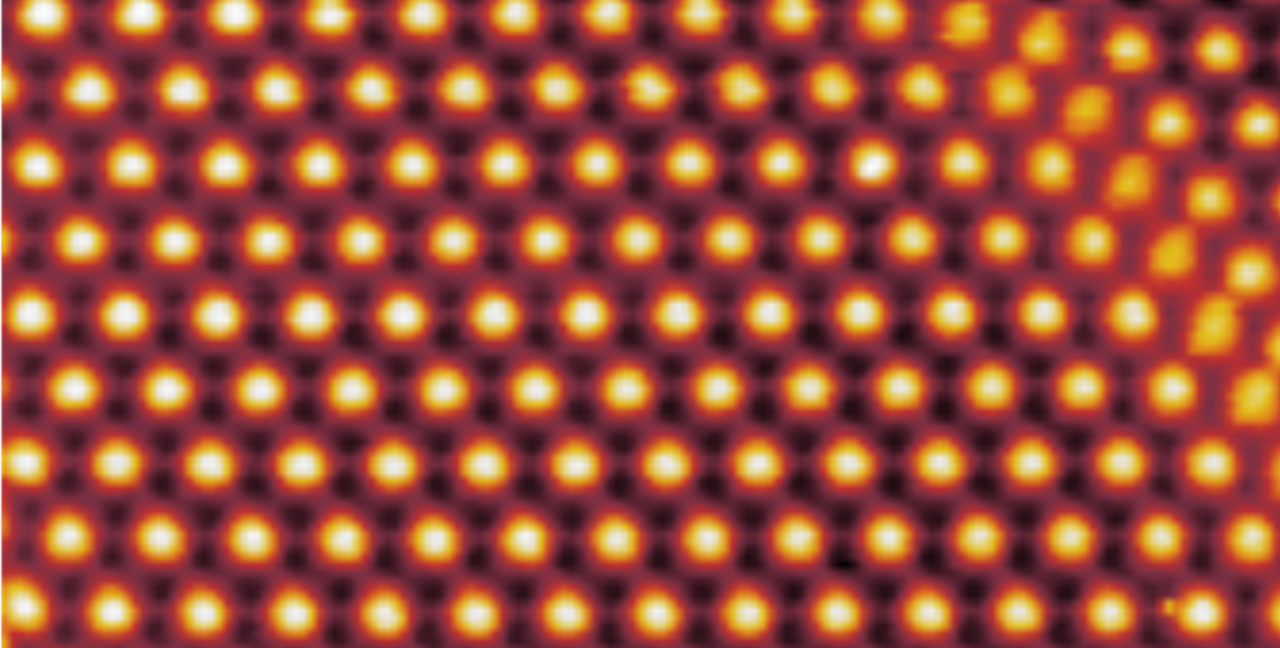
Unusual Superconductivity Observed in Twisted Trilayer Graphene
The ability to turn superconductivity off and on with a literal flip of a switch in so-called "magic-angle twisted graphene" has allowed engineers at Caltech to observe an unusual phenomenon that may shed new light on superconductivity in general.
The research, led by Stevan Nadj-Perge, assistant professor of applied physics and materials science, was published in the journal Nature on June 15.
Magic-angle twisted graphene, first discovered in 2018, is made from two or three sheets of graphene (a form of carbon consisting of a single layer of atoms in a honeycomb-like lattice pattern) layered atop one another, with each sheet twisted at precisely 1.05 degrees in relation to the one below it. The resulting bilayer or trilayer has unusual electronic properties: for example, it can be made into an insulator or a superconductor depending on how many electrons are added.
Superconductors are materials that exhibit a peculiar electronic state in which electrons can flow freely through the materials without resistance—meaning that electricity flows through them without any loss of energy to heat. Such hyper-efficient transmission of electricity has endless potential applications in the fields of computing, electronics, and elsewhere.
However, the catch with superconducting is that in most materials, it takes place at extremely low temperatures, usually only a few degrees above absolute zero (−273.15 degrees Celsius). At such temperatures, electrons forms pairs that behave in a fundamentally different way compared to individual electrons and condense into a quantum mechanical state that allows for electron pairs to flow without being scattered.
Superconductivity was first discovered more than a century ago, yet scientists still do not fully understand the precise mechanisms behind electron-pair formation for some materials. In conventional superconductors, such as metal aluminum, it is well understood that the attraction between electrons that leads to the formation of electron pairs is due to the interaction of the electrons with the material's crystal lattice. The behavior of these materials is described using the Bardeen–Cooper–Schrieffer (BCS) theory, named after John Bardeen, Leon Cooper, and John Robert Schrieffer, who shared the Nobel Prize in Physics in 1972 for the theory's development.
While studying magic-angle twisted trilayers of graphene, Nadj-Perge and his colleagues discovered that superconductivity in this material exhibits several very unusual properties that cannot be described using BSC theory, making it likely also an unconventional superconductor.
They measured the evolution of the so-called superconducting gap as the electrons are removed from the trilayer with the flip of a switch to turn an electric field on or off. The superconducting gap is a property that describes how difficult it is to add or remove individual electrons into a superconductor. Because electrons in a superconductor want to be paired, a certain amount of energy is required to break those pairs. However, the amount of energy can be different for pairs moving in different directions relative to the crystal lattice. As a result, the "gap" has a specific shape that is determined by the likelihood that pairs will be broken by a particular amount of energy.
"While superconductors have been around for a long time, a remarkably new feature in twisted graphene bilayers and trilayers is that superconductivity in these materials can be turned on simply through the application of a voltage on a nearby electrode," says Nadj-Perge, corresponding author of the Nature paper. "An electric field effectively adds or removes extra electrons. It works in a very similar way as the current is controlled in conventional transistors, and this allowed us to explore superconductivity in ways that one cannot do in other materials."
The team established that in twisted trilayers, two superconductivity regimes with differently shaped superconducting gap profiles are present. While one of the regimes can perhaps be explained with a theory that is to some extent similar to BCS, the presence of two regimes shows that within the superconducting phase an additional transition is likely to take place. This observation, alongside measurements taken at various temperatures and magnetic fields, points to the unconventional nature of superconductivity in the trilayers.
The new insights by Nadj-Perge's team give essential clues for the future theories of superconductivity in twisted graphene multilayers. Nadj-Perge notes that it appears that more layers make superconductivity more robust while remaining highly tunable, a property that opens up various possibilities to use twisted trilayers for superconducting devices that may someday be used in quantum science and perhaps quantum information processing.
"Besides its fundamental implications to our understanding of superconductivity, it is remarkable that adding an extra graphene layer made studying superconducting properties easier. Ultimately this is what enabled our findings," Nadj-Perge says.
The paper is titled "Evidence of unconventional superconductivity in twisted trilayer graphene." Co-authors include Jason Alicea, William K. Davis Professor of Theoretical Physics; Caltech graduate students Hyunjin Kim and Youngjoon Choi, leading authors on the paper; graduate students Robert Polski and Yiran Zhang; Cyprian Lewandowski, Moore Postdoctoral Scholar in Theoretical Physics; and Alex Thomson, Sherman Fairchild Postdoctoral Scholar in Theoretical Physics, who is now an assistant professor at UC Davis; and Kenji Watanabe and Takashi Taniguchi of the National Institute for Materials Science in Japan.
This research was funded by the National Science Foundation, the Office of Naval Research, the United States Department of Energy, the Kavli Nanoscience Institute, the Institute for Quantum Information and Matter at Caltech, the Walter Burke Institute for Theoretical Physics at Caltech, and the Kwanjeong Educational Foundation.
Image: The surface of a trilayer of graphene, as imaged by a scanning tunneling microscope. Due to the twist of a second layer, trilayer height is modulated with a period of about 9 nanometers.